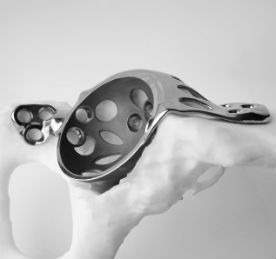
"We get some quite emotive responses: consultants have told us they’ve had patients crying in clinic," reveals Michael Colling-Tuck.
Medical manufacturers might usually keep instances of their products provoking tears under wraps, but JRI Orthopaedics has reason to be proud in this case: the patients to which product manager Colling-Tuck is referring are sufferers of bone loss – due to failed hip-implant surgery or large resections following cancer – and the lachrymose scenes he describes are those of joyful relief, of hope.
"Patients are often completely disabled due to the failure of previous surgeries, and this causes problems with pain and also immobility," Colling-Tuck says. "They’re usually confined to wheelchairs or to their homes, unable to get out, and what these implants tend to do is to enable mobility again and remove pain in many cases. They can restore patients’ quality of life."
What makes JRI Orthopaedics’ implants special and allows surgeons to offer revision procedures previously deemed impossible is the fact that they are completely patient-specific. Designed and 3D printed from a CT scan of the patient’s joint, each implant is able to precisely meet the required shape. This means it can fill the nooks and crannies left vacant by bone fused to – and removed along with – previous implants and can likewise accommodate other difficult-to-remove materials such as old bone cement.
The bespoke implant, known as the AMACE, is also able to overcome another complication that makes standard implants unsuitable, as Colling-Tuck explains.
"Over time, the body tends to respond differently to an implant and the forces acting upon it, so the bone remodels," he says. "What tends to happen is that it creates a build-up of bone in some places but, more importantly, a lot of bone is resorbed in others, and this can then lead to huge defects."
The majority of the standard implants manufactured and available to surgeons are designed to work with primary total hip replacement anatomy that is found in a patient’s first hip replacement, rather than for the complex and differing requirements of revision surgery.
"What we really need is something that is shaped to fill those defects, otherwise you don’t have mechanical structural support so, effectively, you don’t have a stable surface for it to biologically bond with or to support it," Colling-Tuck says. "That’s what we’re looking for when we do revision surgery: stable components."
Joint venture
After a patient has been indentified, a special CT scan is commissioned to ensure that radiographers sufficiently capture residual bone, metalwork and any other foreign materials, such as cement, sitting within the joint area.
This is then shared with JRI’s partners in Belgium, a company named Materialise able to create a 3D digital reconstruction of the patient’s joint, in which each of these aspects – bone, metal and cement – can be separately viewed and manipulated. A design for the proposed implant can then be put together.
"The aim here is always to put the anatomy back to what it was before this bone loss occurred," Colling-Tuck says, "The overall goal is the restoration of the centre of rotation of the hip."
Because the 3D printing method that will be used is additive, engineers are able to create a design that incorporates a lattice structure.
"The clever part of the 3D printing is that, rather than just creating a concentric socket, they’ll be able to make the back of the implant, the convex side, whatever shape is required to fill those defects," Colling-Tuck explains. "That’s really important, because we need to have a lattice structure, a porous coating that’s in contact with the bone, so that the bone will grow on to it."
The ability to create a bespoke design also means the engineers and surgeon are far better equipped to achieve a ‘click-fit’ – that is to say, the implant must sit close enough to existing bone to hold its position with the help of screws until new bone growth, over the course of six to 12 weeks, can bond it securely in place.
Once the implant has been digitally sculpted, the engineers’ proposed design is sent to the surgeon, and a process of back-and-forth consultation ensues to make sure that the final implant will stand up to scrutiny from a mechanical and a clinical perspective.
"Once we’ve got the 3D model, we can do a bone-density analysis to pick out which parts of the bone are thickest and where the optimum screw position is," Colling-Tuck explains. "The company will send that back to the surgeon, and the surgeon will then look at it and say ‘Okay, I understand that, in theory, you’re saying these screws should be directed here but, actually, I think clinically I need them in these positions, and I want to alter the centre of rotation’."
In fact, this interdisciplinary exchange continues even into theatre, with the engineers providing the surgeon with a 20-page booklet outlining the procedure in physical terms, as well as what could be described as an ‘analogue 3D rendering’ of the parts involved. Anatomically accurate plastic versions of the new implant and the patient’s hemi-pelvis, complete with detachable bone, are shared together with the real implant.
"This is fantastic because it means that, before the surgery, the surgeon can actually hold this hemi-pelvis and this plastic implant in their hand, and say, ‘Right, this is how it fits, this is what I need to do, I’m aware that these are going to be my challenges, and I know what bone I need to remove’," Colling-Tuck says.
During the procedure itself, the surgeon can compare the acetabulum with the plastic pelvis to see whether the planned geometry and implant fit is achieved. A plastic screw-position guide also helps ensure the correct screw angle is achieved, all helping lift what Colling-Tuck describes as the "shroud of uncertainty".
The implant itself takes several days to print, created using an additive process in which putting powder is built up to form the finished titanium alloy product.
Primary goal
At present, at three or four times the price of a standard hip, these bespoke implants are available only for revision surgery in patients for whom no other prosthesis would be viable. However, JRI does provide a different kind of bespoke hip for some complex primary anatomy indications in the form of the Unique Hip, a femoral component created using CNC milling, in which the shape is gradually cut from a solid metal block.
As 3D printing gets less expensive in the years to come, Colling-Tuck can foresee a time when all hip implants, whether revision or primary, could be produced using this method on a per-patient basis. And as the design and printing process is fine-tuned, he thinks this could, one day, even happen at source.
"The exciting future might be that we make the implant in theatre, with a scan of a patient, and then we create it on the side, specific to their anatomy, there and then. The machine prints it, and we then implant that in the bone. That’s where we’re aiming our technological research."
JRI has a strong incentive for wanting to be at the forefront of developments: the small Sheffield-based company, owned by Orthopaedic Research UK, reinvests all of its profits in the charity’s research. Against growing international competition in an increasingly commoditised NHS, JRI hopes to secure its prospects by marking itself as a leader in custom and revision hips
Just a little further south in the UK, researchers at Nottingham Trent University (NTU) are applying 3D printing to a new and potentially game-changing technique that could one day see the metal in bone implants replaced by tough, bioresorbable composite materials.
Leading the project is Manolis Papastavrou, a member of the Design for Health and Wellbeing Research Group at NTU’s School of Architecture, Design and the Built Environment (SADBE). He explains the work was prompted by the fact that, despite extensive research over the past 40 years, the realisation of synthetic hard-tissue substitutes for clinical use remains a distant goal.
"This research is driven by the need for synthetic substitutes that exhibit high porosity without it compromising their mechanical properties," he says. "Porosity is important for nutrient transfer and vascularisation, but it has a detrimental effect on the fracture toughness of bioceramic materials."
Hips to be cool
In a project overseen by Professor Philip Breedon from the SADBE and Dr David Fairhurst from the School of Science and Technology at NTU, Papastavrou is trialling a hybrid method of additive manufacturing and a technique known as ice templating, in which the microstructure of a part is controlled by the growth of ice crystals. The system allows a scaffold of synthetic hard tissue to be structured on a macro and micro-scale, helping to improve toughness and load-bearing.
"The element that stands out is the ability to control the microstructure of the struts in the lattice by altering the processing and material parameters of this technique," Papastavrou says.
He believes that the new approach to 3D printing could allow very intricate constructions to be created in a shorter period of time, with structural and compositional gradients able to be built into the scaffolds.
"I envisage this technique being used in the future for fabricating patient-specific implants for large bone defects, ultimately replacing the use of metals, which are often associated with complications due to their high stiffness," Papastavrou says. "The applications could range from complex fractures and joint replacements to reconstructive surgery following the resection of bone tumours.
"The technique offers the ability to tailor the overall geometry of an implant so as to achieve a good fit and mimic the density of the substituted area. Most importantly, it is designed to be used with a wide range of bioresorbable materials."
The next steps will include tests to assess the performance of the 3D-printed structures in vitro as well as fine-tuning the method used, but Papastavrou is hopeful that the first clinical applications could be seen within the next decade.
While engineers in Nottingham and Sheffield take steps to make their visions a reality, it is clear that 3D printing, with its capacity to better replicate the body’s structures, has sculpted itself an integral role in the future of bone implants.