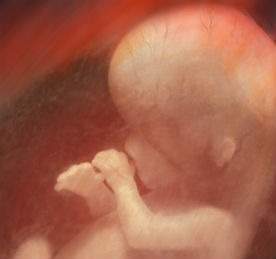
Smoothies, pills and DIY art: the majority of placenta-related headlines typically surround various eccentric uses for the organ after the foetus has been born – but methods of preserving, distributing or consuming placenta are significantly less valuable to science than establishing how it actually functions. The placenta is the only temporary human organ, in the sense that it is created when needed and then discarded. It is therefore notoriously risky to analyse, given that it is only present while a foetus is.
Gestational diabetes, pre-eclampsia, preterm birth and stillbirth are just some of the complications of problems with the placenta. Improving the chances of preventing these outcomes requires substantial further research – which has not gone unnoticed. In 2015, the US National Institutes of Health announced $41.5 million of funding for the Human Placenta Project as part of an initiative to support the development of new technologies to monitor placental health in an effort to improve the well-being of expectant mothers and their babies.
‘Organ-on-a-chip’ (OOAC) research and development is largely still in its infancy. Designed as a multichannel microfluidic cell-culture chip that simulates the functions of entire organs and organ systems, these devices allow scientists to perform intricate and variable analyses of human anatomy. Each device is composed of a flexible polymer that contains hollow microfluidic channels lined with living human cells. They aim to represent human responses to drugs better than living mammals or in-vitro cells in a dish, and are already showing promise in reducing levels of animal testing.
Great impression
In 2011, Professor Shoji Takeuchi from the University of Tokyo teamed up with Dr Shigenori Miura to begin culturing cells in microfluidic devices. "Until now, we have not had a proper device that can analyse material flow through the placenta under flow conditions," explains Takeuchi. "Our microfluidic device can provide flow conditions that enable us to analyse cellular responses that may affect material transfers."
Takeuchi’s research team in Tokyo consequently created a multilayer microfluidic device that imitates the structure of the placental barrier – the wall that supports blood flow, oxygen and material exchange between mother and foetus. Using this device, they discovered that fluid shear stress (FSS) – shear forces caused by fluid flow – in the mother’s body stimulates the development of microvilli in epithelial cells in the placenta. The placental barrier is one example of a tissue that has adapted to multiple stimuli.
Compared with conventional static culture methods, in which forces such as fluid flow are not used, applying the external mechanical cue of FSS induces formation of microvilli on placental epithelial cells and controls cell function.
In terms of the OOAC, physics can be as fundamental as biochemistry and genetics when it comes to mimicking human physiology. Human cells from virtually any organ can be grown in a laboratory using microfluidics and other engineering techniques to imitate the human body, reproducing the physiology, biochemistry and microanatomy of the true organ.
By recreating the placenta’s barrier functions at the size of a computer chip, Takeuchi’s research offers unique insight into the mysterious organ’s workings – the microdevices are translucent so provide a window into the inner functions of human organs. With little research having been previously conducted on maternal blood flow to the placenta, Takeuchi’s findings are significant contributors to placental transfer analysis.
Generally, expectant mothers should avoid taking medicines as they can affect the normal development of the baby. In fact, some drugs are harmful to the foetus’s health and can cause malformation when they reach it through the placental barrier.
"We hope our device may improve the screening of drugs useful to expectant mothers," says Takeuchi. "If we could know which drugs are permeable to this barrier, we can design ones that are not. Thus, pregnant women will be able to take medicines more safely."
This is especially pertinent for pregnant women who cannot safely stop taking medication due to a health disorder or irregularity of their own.
Traffic monitoring
Placental barrier cells are constantly exposed to shear forces from the flow of maternal blood to the placenta. However, little research has been conducted on the effect of this flow on cell morphology and function. When the placenta doesn’t function correctly, the health of mother and baby suffers. A puzzling and significant question for scientists is how the placenta manages, by reacting to various stimuli, to import some substances and block others. If an answer is found, it could drastically improve pregnancy outcomes.
Another high priority for the placenta-on-a-chip’s development is teratogenicity testing to investigate toxic effects on the unborn child – effects that can jeopardise the health of the mother as well as the baby. As well as supplying oxygen and nutrients to the foetus, the placenta helps expel waste and stop harmful environmental exposures, like bacteria, viruses and certain medications, from reaching the baby.
In order to analyse this traffic of substances across the placental barrier, Takeuchi and his researchers tracked the movement of glucose through their device.
"Cells get their energy from glucose, so its presence is vital for foetal growth. Due to this importance, we ensured the localisation pattern of the glucose transporter in the human placental barrier was well investigated," explains Takeuchi. "So we knew if the localisation pattern in our device was similar to that in the placental barrier tissues, then our device was good – at least in this aspect."
"Mechanical stress is an important cue that regulates cellular morphology and tissue structure," continues Takeuchi. "We found that TRPV6, a calcium ion channel present on the cellular membrane of the placental barrier cells, played a critical role in inducing the in-vivo-like cellular structure of the placental barrier cells – the microvilli – in our device."
In order to reconstruct organ-level tissue function in vitro, scientists should implement this ‘mechanical stimuli’ characteristic in the tissue of interest. They may then be able to generate or regulate tissue functions by properly controlling the mechanical stimuli.
The mechanics of biology
There are a number of tissues and cells possessing a biological system that can sense and respond to mechanical stress. For example, vascular endothelial cells have specific molecules that sense FSS generated by blood flow. And Takeuchi still has designs on perfecting his placental-barrier device.
"One of the important issues in reconstructing the human placental barrier is the cell source to be used," he points out. In the placental tissue, the barrier is composed of the epithelial cell layer of the placental villi, vascular endothelial cells from the foetus and the extracellular matrix, which contains collagen. It is possible to obtain human vascular endothelial cells from a human umbilical cord and successfully implement them in the device’s barrier construct.
"However," explains Takeuchi, "it is very difficult to isolate and culture the epithelial cells of the human placental villi because they form a fused cell layer due to cell fusion in the tissue.
"So, in our device, instead of using primary cells from the placental barrier, we now use BeWo cells, which are the established cell line derived from human choriocarcinoma [a malignant uterine tumour that originates in the outermost layer of the embryonic membrane]. Since tumour cells cannot stop cellular proliferation, it is hard to keep the layer of epithelial cells in the device for a long time, which may be critical for practical use."
However, recent research has shown that epithelial cells of the placental villi can be obtained from human embryonic stem cells after the suitable induction of the cellular differentiation.
"Therefore, we believe that if the human placental barrier is successfully reconstructed by combining the differentiated human embryonic stem cells and the human vascular endothelial cells in the device," continues Takeuchi, "then it will be suitable for continuous use and will provide more reliable data than the device with the BeWo cell construct."
So what does this mean, going forward, for pregnant mothers and mechanobiological research? The interesting question is whether these insights can be transferred from the microchip and applied practically to the delicate real-world balancing act of actual pregnancy. But, at the very least, by optimising a hybrid of living organisms and mechanical engineering, Takeuchi is opening up a new research frontier that promises to deliver vital biomedical insight for natal care.