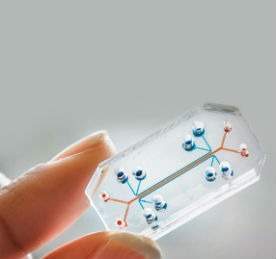
It doesn’t take an activist to tell you that animal testing in drug development is, if nothing else, grossly inefficient. Innumerable lives are lost in this process, yet about 85% of therapies fail in early clinical trials – and of those that do make it to phase three, only half are approved. Animal models are seen as a necessary evil right now, but the pharmaceutical industry desperately needs alternative ways to screen candidates in the lab – ways that more accurately predict human responses.
In early studies, new compounds are often tested on cultured human cells, but these have limitations too: they’re hard to keep alive for very long, and they don’t usually represent human physiology sufficiently for scientists to rely on the data without backing it up with information from animals.
But soon, a device that aims to better represent our responses to drugs – better than living mammals and cells in a dish alike – could be implemented. Enter Professor Don Ingber and his team at the Wyss Institute of Biological Inspired Engineering at Harvard University.
Multi-award-winning Ingber is said to have catalysed the convergence of far-reaching disciplines ranging from biology to computer science, to architecture. His mission is to identify the mechanisms living organisms use to self-assemble and apply these design principles to novel medical devices.
The organ-on-chip origin
In recent years, Ingber and colleagues have been engineering plastic microchips that represent the structure and function of living organs, like the lung, heart and intestine. The team calls its devices organs-on-chips, and their work has just been honoured with the 2015 Design of the Year award from London’s Design Museum.
The project was nominated by Paola Antonelli, New York Museum of Modern Art’s senior curator of architecture and design, who called the project "the epitome of design innovation – elegantly beautiful form, arresting concept and pioneering application".
Each organ-on-chip is composed of a flexible polymer that contains hollow microfluidic channels lined by living human cells. The microdevices are translucent, providing a window into the inner workings of human organs.
Crucial to the work is the theory that physics can be as fundamental as biochemistry and genetics when it comes to mimicking human physiology. Since the 1970s, Ingber has been working on the idea that mechanical forces are vital for controlling development.
After collaborating with renowned scientist George Whitesides, he learnt that by applying a process used in computer chip manufacturing called graphic etching, it was possible to make tiny hollow channels with inlets and outlets inside the devices.
"We basically started to view those channels as little micro blood vessels or vascular networks," Ingber reveals. "We integrated them to develop culture environments that would allow cells to be exposed to fluid change and physical forces that could reconstitute the normal physical environment."
Importantly, the team found that through adding porous membranes to the chips, they could have one cell type on one side and another cell type on the other. This is how organs (which are essentially simply two or more different tissues working together) do their jobs.
Even cells that are particularly tricky to keep alive fare better in chip form, thanks to mechanical forces. Ingber gives the example of colon cells used frequently in drug development, called Caco-2s.
"If you take Caco-2 cells, they aren’t very differentiated in culture. They’re pretty flat," he says. "But if you put them in a chip, and you give them flow and peristalsis-like motion, they spontaneously form villi like your intestine. It’s really quite amazing."
New developments
The first device the group successfully completed was the lung-on-chip in 2010. Ingber says it took about a year and half to get the product working before the team could submit their paper on the project. It was developed by placing two layers of living tissues – the lining of the lung’s air sacs and the blood vessels surrounding them – across a porous flexible boundary. Air can then be delivered to the air sac cells, while a rich culture medium flows into the capillary channel to mimic blood. But the real challenge was getting the chip to simulate breathing.
To solve this, inspired by how breathing works in a human lung, the team employed the use of a vacuum. In real life, when our chest expands, we create a void that sucks air into the lung and causes the air sac walls to stretch.
"With the lung-on-chip, we found that if you added particulates but you didn’t have breathing motions, you didn’t get toxicity. That had never been seen before," says Ingber. "We were able to confirm it in animal models."
Since the lung device was developed, nine other chips have been introduced, mimicking practically everything from the gut to bone marrow. Some have been harder to produce than others, Ingber says, but in general, a year and a half seems to be the time it takes for most of them to pass muster. He does admit, though, that working on a blood-brain barrier device has been difficult.
"We’re getting close. For the most part, we’ve got them to work pretty quickly, but demonstrating that it really represents unit-level function takes time. Getting the device to be robust takes time."
He points out that there are some things the chips won’t be suitable for, like representing consciousness or depression. The group isn’t able to mimic brain activity at this point, though it might be possible to model neural injury in the future.
"Most things are approachable, but some of them take decades to get there," Ingber says. "But we’ve got most of the organs – most of the physiological systems are represented already."
The chips are well on their way to commercial success. In January, the team founded a start-up called Emulate, which is working with corporate pharmaceutical, biotechnology and cosmetic organisations. It was recently announced that Janssen Biotech, a subsidiary of Johnson & Johnson, will use the organs-on-chips in a new research collaboration. The devices will be deployed across Janssen R&D programmes in drug design and selection. The lung-on-chip and a thrombosis-on-chip will be used for evaluating pulmonary thrombosis, while a liver-on-chip will be applied towards better predicting toxicity – a major cause of drug failure in the clinic.
"We’ve developed a blood clot in a lung-on-chip, and that’s something they’ve found to really mimic things they’ve seen before in vivo with pretty high validity, but it’s something they couldn’t model before," says Ingber. "I think that’s a great testament to how this will work."
He reveals that a study is also underway with pharmaceutical giant AstraZeneca to model the liver of different species – essentially developing an animal version of the liver-on-chip. The resulting devices will be tested alongside the human models to further understand the extent to which drug safety results in animals can predict how a therapeutic candidate might impact people.
"Drug companies commonly do tests in dogs and rats and get completely conflicting results, and then they have to figure out what they think will happen in humans," says Ingber. "And human liver cells are only good for about three days before they die. But now, we can keep them alive for weeks, maybe, and they’ll actually be highly functioning. If we could show that the chips mimic human response better than the dog and rat, that would be a really good proof of principle, I think."
Chipping into the future
The next stage of Ingber’s work is to couple multiple chips in order to more closely mimic full human physiology, where organs respond to each other. He reveals that Emulate is trying to commercialise an instrument that can make this coupling process possible.
"By next year, we need to show we can keep seven organs-on-chips alive for three weeks. And the year after we achieve that, we need to show we can do ten organs for a month."
Aside from reducing animal testing in drug development, the chips could have implications in personalised medicine, or be used to indicate a subgroup that might benefit from a particular pharmaceutical.
"Pharma companies spend billions of dollars to take a drug from bench to patient," adds Ingber, "and when they do the clinical trial, it takes thousands of patients and many years, and it will often fail. But they’ll go back and do a statistical analysis to try and find a subgroup that might have responded better. They’ll do a smaller trial, and if they’re lucky, they’ll get efficacy. With organs-on-chips and personalised medicine, you can make an organ and find a genetic marker within it that might have susceptibility to a particular condition. And then you can develop drugs for just those people."
And it could get even more personal than that, he reveals. It is hoped that induced pluripotent cells (a type of stem cell that can be generated from adult cells) from patients will one day be used in hospitals to predict drug efficacy. As the culture doesn’t reconfigure organ function alone, however, it might not be the most accurate predictor of whether a therapy will prove efficacious. "But if you put them in a chip, they might."
He theorises that in the future, you might get a special organ-on-chip made for patients to predict the best treatment for their condition. It means a drug could be non-invasively tested on your own lung or intestine – not a dog’s or a rat’s, or that of the average person doing a trial.
Clearly excited about the potential of his work, Ingber is adamant that in order to practice good science, effective design is crucial. He hopes the devices will hit the mainstream in the next two years – and if their use proves fruitful, some animal models could start to be eliminated. "We’re not replacing all animal testing, but you can really reduce the numbers for various tests," he stresses.
He’s not saying lab rats will soon be a thing of the past, exactly. But thanks to scientists like Ingber and his team, use of them could soon be significantly reduced.